Review Article / Open Access
2021; 4(3): 425 - . doi: 10.31488/HEPH.165
Sirolimus for Treatment of β-Thalassemia: From Pre-Clinical Studies to the Design of Clinical Trials
Maria Rita Gamberini*1, Marco Prosdocimi2, Roberto Gambari3
1. Thalassemia Unit, Arcispedale S. Anna, Ferrara, Italy
2. Rare Partners Srl Impresa Sociale, Milano, Italy
3. Department of Life Sciences and Biotechnology, University of Ferrara, Italy
*Corresponding author: Maria Rita Gamberini, Thalassemia Unit, Arcispedale S. Anna, Ferrara, Italy Roberto Gambari, Department of Life Sciences and Biotechnology, Ferrara University, Ferrara, Italy
Abstract
Beta-thalassemia is a rare disease in EU and in USA. Even if prognosis is improved, life expectancy is still shortened and, in most cases, patients must receive continuous blood transfusions to survive. Induction of fetal hemoglobin (HbF) has been suggested as a promising strategy to ameliorate the clinical status of the β-thalassemia patients. Among HbF inducers, the repurposed drug sirolimus was found promising in β-thalassemia patients and sickle-cell disease (SCD) patients, since it has been found as a potent HbF inducer in vitro on the K562 experimental system, ex vivo on erythroid precursor cells (ErPCs) from β-thalassemia patients, in vivo in experimental SCD mice and in vivo on a limited number of SCD patients. For these reasons sirolimus obtained the Orphan Drug Designation by the European Medicinal Agency (EMA, Europe) and by the Food and Drug Administration (FDA, USA) for both β-thalassemia and SCD. These research background and technological transfer activity allowed the design of the ongoing NCT03877809 (A Personalized Medicine Approach for β-thalassemia Transfusion Dependent Patients: Testing sirolimus in a First Pilot Clinical Trial) and NCT04247750 (Treatment of β-thalassemia Patients with Rapamycin: From Pre-clinical Research to a Clinical Trial) clinical trials, both based on the use of low-dosages of sirolimus for a 12 months period.
Keywords: Sirolimus, β-thalassemia , fetal hemoglobin, clinical trials
Introduction
Beta-thalassemia, one of the most common inherited hemoglobinopathies worldwide, is due to autosomal mutations in the gene encoding β-globin, which induce an absence or low-level synthesis of this protein in erythropoietic cells [1-3]. The phenotypes range widely from asymptomatic (β-thalassemia trait or carrier) to clinically relevant anemia which is categorized as transfusion-dependent β-thalassemia (including thalassemia major) and non-transfusion-dependent β-thalassemia (thalassemia intermedia).
About 80 to 90 million people (~ 1.5 % of the global population) are carriers of β-thalassemia with approximately 60,000 symptomatic individuals born annually [4]. The annual incidence of symptomatic individuals is estimated at 1 in 100,000 worldwide and 1 in 10,000 in the European Union (EU) [5]. Incidence is highest in the Mediterranean region, the Middle East, and South East Asia (particularly India, Thailand, and Indonesia; this region accounts for approximately 50% of affected births), but incidence is increasing worldwide (eg, Europe, the Americas and Australia) as a result of migration [6,7].
Worldwide, nearly 300 mutations around the β-globin gene have now been reported to cause -thalassemia [8]. Most are point mutations either in the gene or its immediate flanking regions, but some are caused by small deletions removing all or part of the β-globin gene. Some rare β-thalassemias are caused by deletions removing the upstream regulatory elements (collectively referred to as the β-globin locus control region), leaving globin genes intact. The mutation associated with the very common structural hemoglobin variant hemoglobin E also leads to abnormal, inefficient splicing of the associated β-globin gene, resulting in a phenotype of β-thalassemia [9,10].
Depending on the degree of quantitative reduction in the synthesis of normal β-globin, β-thalassemia mutations are classified into three groups: (1) β0-thalassemia mutations, causing the absence of β-globin; (2) β+-thalassemia mutations, severely reducing the output of β-globin; and (3) β++thalassemia mutations (known as silent β -mutations), mildly reducing the output of β-globin [8].
The pathophysiology of anemia is linked with the impaired production of α-globin chains leading to an unbalanced excess of -globin chains; in erythroid cells free -globin molecules are highly unstable and undergo auto-oxidation, forming α-hemichromes (α-globin monomers that contain oxidized ferric iron) and reactive oxygen species (ROS) which trigger cascades of events leading to hemolysis of mature red blood cells and destruction of immature erythroid precursors in the bone marrow (ineffective erythropoiesis).
In β-thalassemia the clinical picture is directly related to the degree of imbalance between α-like and β-like globin chain synthesis in erythroid cells and the causative β-globin mutation has the largest impact on disease severity. Homozygotes or compound heterozygotes for two β0-thalassemia alleles cannot produce any HbA (α2β2); such individuals generally have the most severe anemia and their survival depends on blood transfusions performed from early age for life (thalassemia major). However, there is also remarkable clinical variability, even in patients who inherit identical β-globin genotypes. Copy number variations of α-globin genes and variations affecting fetal hemoglobin (HbF, α2γ2) production are the two best-characterized modifiers of severity in β-thalassemia, both affecting the central mechanism of underlying disease pathophysiology (ie, the degree of globin chain imbalance and the excess of α-globin chains).
In this respect, the number of functional α-globin genes has a clear direct effect on the clinical severity of patients with β-thalassemia. Coinheritance of extra α-globin genes with heterozygous β-thalassemia pushes the globin chain imbalance further, converting a typically clinically asymptomatic state to thalassemia intermedia. In this case, the severity of anemia depends on the number of extra α-globin genes and the type of the β-thalassemia alleles. Individuals who have coinherited α-thalassemia have less redundant α-globin and tend to have less severe anemia. The degree of amelioration depends on the severity of the β-thalassemia alleles and the number of functional α-globin genes. Several family, cohort, and case-control studies have reported that the coinheritance of α-thalassemia ameliorates the severity of β-thalassemia. These studies have shown that deletion of one or two α-globin genes (-α/αα, --/αα, or - α/- α) is associated with a milder disease in most patients with β-thalassemia and in some circumstances transforms the phenotype from transfusion-dependent thalassemia to thalassemia intermedia.
Accordingly, therapeutic downregulation of α-globin expression is a new strategy to counteract the α/β globin imbalance and some approaches using genome editing are proposed in preclinical studies [11,12].
As far as variations affecting fetal hemoglobin levels, the production of HbF after birth is an important factor in modifying the clinical severity, because an increased γ-globin level will bind the additional α-globin and form HbF. Thus, the increased γ-globin production decreases the α/β chain imbalance, improves the ineffective erythropoiesis, decreased hemolysis, increases the total hemoglobin levels as consequence of the improved survival of red cells containing higher levels of HbF.
Many factors are involved in setting the Hb F level; some are located in the β-globin gene cluster and others on different chromosomes. Three quantitative trait loci associated with increased production of γ-globin and HbF have identified in several molecular, genetic linkage and genome-wide association studies. The Xmn1-HBG2 polymorphism in the β-globin gene cluster, polymorphisms in the HBS1L-MYB intergenic region on chromosome 6q23 and the BCL11A enhancer on chromosome 2p16 have all been shown to increase γ-globin gene expression [13]. Recently, it was also shown that certain mutations of the KLF1 gene increase the production of HbF, which in turn ameliorates the clinical severity of β-thalassemia [14].
The earliest clinical observations suggesting the advantageous role of HbF in thalassemia came from patients with rare forms of β-thalassemia, particularly those with large deletions responsible of δβ0-thalassemia or hereditary persistence of fetal hemoglobin (HPFH), with absence of β-globin production but associated to high quantity of γ chain production, resulting in high levels of HbF with a relatively benign clinical course [8,15]. More-recent clinical studies have disclosed that the naturally higher production of Hb F improves the clinical course in a variety of patients with β-thalassemia [13-21]. Accordingly, these observations have promoted research for inducers of HbF that can therapeutically reproduce what occurs in β-thalassemia patients with natural persistence of higher levels of HbF [22-24]. Furthermore, approaches using genome editing are available finalized to induction of HbF following elimination of genomic sequences encoding for transcriptional repressors or genomic sequences targeted by these regulatory factors [25-29]. In this context clinical trials are occurring, such as NCT03655678 (A Safety and Efficacy Study Evaluating CTX001 in Subjects With Transfusion-Dependent β-Thalassemia), based on the use of autologous CRISPR-Cas9 Modified CD34+ Human Hematopoietic Stem and Progenitor Cells (hHSPCs) using CTX001 [30].
Induction of HbF using the Repurposed Drug Sirolimus: a Possible Strategy for -Thalassemia
Table 1. Examples of clinical studies and using sirolimus and rapalogs
Pathology | Drug | Major conclusions | References |
---|---|---|---|
Kidney transplantation | Sirolimus; everolimus | This study examined the evidence of the use of sirolimus and everolimus in (a) prevention of immune dysfunction and renal function preservation in de novo renal transplantation; (b) chronic dysfunction of the renal graft; (c) cardiovascular effects; (d) de novo post-transplant diabetes, and (e) de novo tumor pathology. | Kahan, 1997 [32]; Vasquez, 2000 [33]; Hernández et al., 2011 [34] |
Cardiac transplantation | Everolimus | Everolimus may potentially reduce late term complications, including coronary allograft vasculopathy (CAV), while maintaining the low cellular rejection rates seen with standard therapy. | Schaffer and Ross, 2010 [35] |
Liver transplantation | Everolimus | Everolimus combined with low-dose calcineurin inhibitors (CNIs) decreases the risk of acute rejection. | Tang et al., 2015 [36] |
Lupus erythematosus (SLE) | Sirolimus | A total of 9 studies comprising 145 patients were identified. Sirolimus was promising and well-tolerated in the treatment of SLE. | Ji et al., 2020 [37] |
Lymphangioleiomyomatosis (LAM) | Sirolimus; everolimus | Fourteen prospective studies were enrolled in this systematic review. Sirolimus and everolimus were recommended for the treatment of LAM because they could stabilize lung function and alleviate renal AML. | Wang et al., 2020 [38] |
Tuberous sclerosis complex | Sirolimus; everolimus | Three placebo-controlled studies with a total of 263 participants were included in this study. Evidence was found that oral everolimus significantly increased the proportion of people who achieved a 50% reduction in the size of sub-ependymal giant cell astrocytoma and renal angiomyolipoma. | Sasongko et al., 2016 [39] |
Recurrent meningioma | Everolimus | A total of 20 patients were enrolled. The combination of everolimus and octreotide was associated with clinical and radiological activity in aggressive meningiomas and warrants further studies. | Graillon et al., 2020 [40] |
Pancreatic neuroendocrine tumours (NET) | Everolimus | Everolimus should be recommended as the first line therapy for patients with symptomatic malignant unresectable insulin-secreting pNETs, to control the endocrine syndrome regardless of tumour growth. | Gallo et al., 2017 [41] |
Advanced Differentiated Thyroid Cancers | Sirolimus | The combination of sirolimus, with a well-known cytotoxic agent, cyclophosphamide, provides a well-tolerated and promising alternative treatment for advanced, differentiated thyroid cancers. | Manohar et al., 2015 [42] |
Advanced breast cancer | Everolimus | Everolimus, in combination with exemestane, is proposed for patients with advanced hormone receptor-positive/HER2-negative breast cancer. | Hortobagyi, 2015 [43] |
B-cell lymphomas | Everolimus | A large Phase II study in relapsed/refractory diffuse large B-cell lymphoma (DLBCL) confirmed the substantial activity and good tolerability of everolimus, with thrombocytopenia being the main toxicity. The combination of everolimus and rituximab showed encouraging results, without increasing toxicity. | Merli et al., 2015 [44] |
Metastatic renal cell carcinoma | Everolimus | A Phase III trial demonstrated superiority at interim analysis for everolimus over placebo in patients with metastatic renal cell carcinoma (mRCC). | Motzer et al., 2010 [45] |
Sirolimus, also known as rapamycin [31] is a lipophilic macrolide isolated from a strain of Streptomyces hygroscopicus found in a soil from Easter Island (known by the inhabitants as Rapa Nui). Quite interestingly, the name of its target, mammalian target of rapamycin (mTOR), recalls exactly the name of the substance originally described as rapamycin. Inhibitors of mTOR are used as immunosuppressant, in particular in the setting of prevention of transplant rejection. The mTOR signaling pathway is a central modulator of cellular responses to environmental changes in nutrients and oxygen status, and has been implicated in the regulation of cell growth, translation, autophagy, cytoskeletal rearrangements, and cell survival. The dysregulation of the pathway is implicated in several diseases, particularly neoplastic disorders; therefore, an inhibitor of mTOR like sirolimus not unexpectedly has a number of useful and potentially harmful actions.
The drug is a well-known agent, used for many years for other indications [32-45] (Table 1). For instance, sirolimus or sirolimus-related compounds (collectively summarized as rapalogs) have been employed for treatment of kidney transplantation [32-34], cardiac [35] and liver [36] transplantation, lupus erythematosus (SLE) [37], lymphangioleiomyomatosis (LAM) [38], tuberous sclerosis complex [39], recurrent meningioma [40], pancreatic neuroendocrine tumors (NET) [41], advanced differentiated thyroid cancers [42], advanced breast cancer [43], B-cell lymphomas [44], metastatic renal cell carcinoma [45].
In respect to clinical management of -thalassemia patients, sirolimus was considered a repurposed drug when the first evidence of its ability to induce in vitro HbF production in erythroid precursor cells (ErPCs) from -thalassemia and sickle cell disease (SCD) patients was reported and further validated [46-49] (Figure 1).
The relevance of these reports is due to the fact that no treatment has been so far approved concerning HbF inducers in thalassemia. While hydroxyurea (HU) is frequently used (despite the lack of formal approval), its use is limited by the potential adverse effects and reported efficacy in only a subset of patients [50-53]. Thus, a substantial percentage of thalassemia patients are not treated with HU and may benefit by other treatments. Moreover, some patients become HU resistant after long term treatment.
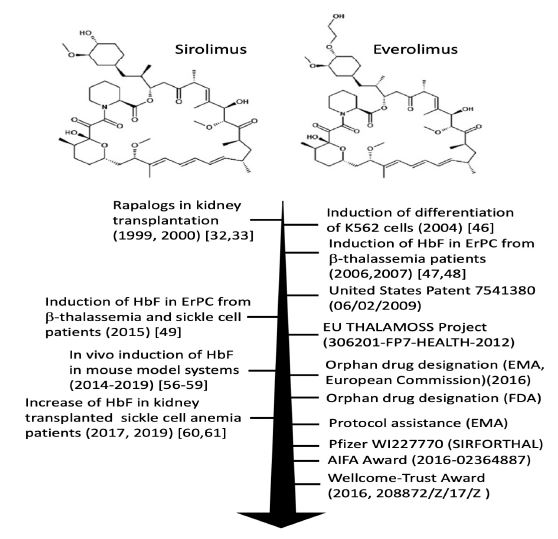
Figure1:Summary of the laboratory research achievements and technological transfer activity leading to the design of the NCT03877809 and NCT04247750 clinical trials
As for the mechanism of action of sirolimus and rapalogs in HbF induction, Bianchi et al. [54] and Finotti et al. [55] pointed out that the mammalian Target of Rapamycin (mTOR) is a key regulator of erythroid differentiation and globin gene expression. A very high number of studies have been published on mTOR; mTOR belongs to the phosphoinositide 3-kinase (PI3K)-related kinase (PIKK) family and forms two distinct complexes (mTORC1 and mTORC2). Rapamycin interacts with FK506-binding proteins, such as FKBP12, with high affinity and the resultant complex interacts with mTOR in the context of mTORC1 to allosterically inhibit mTORC1 activity. This is expected to cause deep alteration in several cellular pathways, since the mTOR signaling pathway is a central modulator of cellular responses to environmental changes in nutrients and oxygen status, and has been implicated in the regulation of cell growth, translation, autophagy, cytoskeletal rearrangements, and cell survival.
As far as the role of mTOR on erythroid cells, it was found that sirolimus-mediated erythroid induction is associated with hypophosphorylation of -p-S6 ribosomal protein and 4E-BP-1. Furthermore, inactivation of both 4E-BP1 and p70-S6K are sufficient steps, since induced differentiation when these molecular events were simultaneously produced by a mixture of drugs involved in downstream alterations of the FRAP-mTOR signal transduction pathway [54,55]. Interestingly, other HbF inducers (i.e. mithramycin, which targets the mTOR regulator raptor) inhibit the mTOR pathway specifically. Finally, HbF induction can be potentiated by siRNAs targeting mTOR, raptor and other member of the mTOR pathways or following transfection of erythroid cells with microRNAs regulating the mTOR pathway (for instance miR-210) [54].
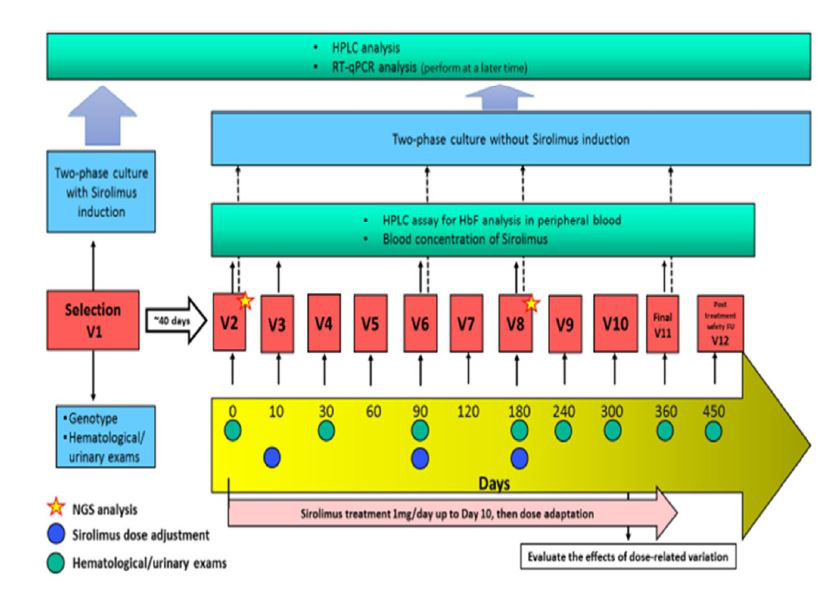
Figure2:Flow chart summarizing the NCT03877809 and NCT04247750 clinical trials
The working hypothesis in using sirolimus for -thalassemia is that the reported sirolimus-dependent increase of the HbF levels will improve the clinical status of the -thalassemia patients, with the aim of reducing the transfusion need in transfusion dependent patients, the majority of the population present in Europe. As far as transfusion independent patients, sirolimus effects should be studied with end points partly different from the ones we will describe in this manuscript.
In conclusion, in vitro data indicate that sirolimus can be repurposed for treatment of -thalassemia for the following reasons: (a) sirolimus increases HbF in cultures from -thalassemia patients with different basal HbF levels; (b) sirolimus increases the overall Hb content per cell; (c) sirolimus selectively induces -globin mRNA accumulation, with only minor effects on -globin and -globin mRNAs; (d) there is a strong correlation between the increase induced by sirolimus of HbF and the increase in -globin mRNA content. Taken together, these data suggest that sirolimus may be an interesting candidate for a role in the treatment of patients with -thalassemia.
In vivo studies using Sirolimus on Animal Model Systems: from In Vitro Evidences to In Vivo Validation
Several studies supported the possible effects of sirolimus in vivo using animal model systems. In 2014, Zhang et al. published a paper providing important contributions to our knowledge of redox and metabolic regulation of erythropoiesis [56]. Among other experiments, they also studied rapamycin treatment in mice, using intraperitoneal administration of 4 mg/kg body weight of rapamycin during five consecutive days/week for 2 weeks in an experimental model of thalassemia. These mice, known as Th3/+, are considered a good model to study ineffective erythropoiesis. In vivo, sirolimus treatment increases red blood cell counts and hemoglobin levels, supporting the concept that sirolimus administration may be of help in patients with ineffective erythropoiesis.
In a model system with sickle-cell phenotype, Khaibullina et al. hypothesized that rapamycin would increase HbF levels. Rapamycin significantly increased γ-globin mRNA and HbF levels [57]. In another report Wang et al. determined whether mTOR inhibition would improve anemia in sickle cell disease (SCD) [58]. Mice with SCD were treated with the dual mTORC1/2 inhibitor, INK128. One week after daily oral drug treatment, erythrocyte count, hemoglobin, and hematocrit were all significantly increased while reticulocyte counts were reduced. These parameters remained stable during 3 weeks of treatment. Similar effects were observed following oral treatment with sirolimus. Sirolimus treatment prolonged the lifespan of sickle cell erythrocytes in circulation, reduced spleen size, and reduced renal and hepatic iron accumulation in SCD mice [58].
More recently Lechauve et al. [59] pointed out an alternative mechanism as the cause why sirolimus improves the situation in mice with thalassemia and why the drug should be studied in patients. Indeed, inhibition of mTOR can activate ULK1 and this mechanism may be of utmost importance. Thalassemic mice treated with sirolimus showed a significant reduction in α-globin accumulation and ineffective erythropoiesis along with a longer life span for red blood cells, a combination of effects potentially important for patients [59].
Case Reports Sustaining In Vivo Activity of Sirolimus in Inducing HbF: Clinical Supports to Laboratory-Based Studies
The in vivo effects of mTOR inhibitors on humans were approached by studying the effects on HbF production in patients treated with rapalogs who underwent kidney transplantation. In a fist study Gaudre et al. reported the case of a kidney transplant recipient with SCD who was treated with everolimus, a mammalian target of rapamycin inhibitor. At 10 months after initiating therapy, the patient's HbF level was dramatically increased (from 4.8% to 15%) and there was excellent tolerance to treatment. Even if this should be considered a case-report study, it supported the concept that rapalogs might be able to induce an increase of HbF production in vivo [60]. More recently, Al-Khatti, et al. observed a significant increase in HbF level after treatment of SCD patients with sirolimus. In addition, there was further increment in HbF level when sirolimus and hydroxycarbamide were combined. This is the first report documenting an increase in HbF after treatment with sirolimus in patients with SCD [61].
On the basis of the published information about the effects of sirolimus on HbF, Orphan Drug Designation (ODD) has been obtained for this repurposed drug by the European Medicinal Agency (EMA) for the treatment of β-thalassemia (code EU/3/15/1585) and of SCD (code EU/3/17/1970). ODD of sirolimus as HbF inducer in β-thalassemia and SCD was also obtained by the U.S. Food and Drug Administration (FDA).
The Sirolimus-Based NCT03877809 and NCT04247750 Clinical Trials
This communication is related on two ongoing clinical trials conducted on ß-thalassemia patients, NCT03877809 (A Personalized Medicine Approach for β-thalassemia Transfusion Dependent Patients: Testing sirolimus in a First Pilot Clinical Trial) and NCT04247750 (Treatment of β-thalassemia Patients with Rapamycin: From Pre-clinical Research to a Clinical Trial). These two trials are based on the use of low-dosages of the repurposed drug sirolimus (rapamycin) for a 12 months period.
The main objective of these interventional, pilot, open-label phase II studies with sirolimus in patients with β-TDT (transfusion-dependent thalassemia) was to verify its efficacy as in vivo HbF inducer aiming to the reduction of the transfusions need with an overall good tolerability. In addition, the possibility to know genotypes and DNA polymorphisms (including HbF-associated polymorphisms) is expected to bring important information for a possible personalized and precision medicine approach in β-TDT.
Study Setting
The first protocol (EudraCT n° 2018-001942-33, NCT 03877809) identified as SIRTHACLIN, is a single centre trial conducted at the Thalassemia Centre of Azienda Ospedaliera-Universitaria S.Anna (Ferrara, Italy). In this case, the β-thalassemia patients will be recruited among patients with β+/β+ and β+/β0 genotypes.
The second protocol (EudraCT n°2018-001469-18, NCT 04247750) identified as THALA-RAP is a multicenter study conducted in Ferrara (Azienda Ospedaliera-Universitaria S.Anna, Ferrara, Italy), Firenze (Azienda Ospedaliera-Universitaria A.Meyer, Firenze, Italy) and Pisa (Azienda Ospedaliera-Universitaria Pisana, Pisa, Italy). In this case, the β-thalassemia patients will be recruited among patients with β0/β0 and β0/β+ genotypes.
The Study Plan, Study Population and Eligibility Criteria
The two phases of the studies, including the period of enrollment and selection of patients (40 days) and the period of treatment (360 days) are reported in Figure 2. The study population of these two clinical trials are adult patients with transfusion-dependent β-thalassemia (TDT) regularly attending the clinical Centres since at least 6 months. Patients will be screened in order to ensure having a sufficient number of patients positive ex vivo to the treatment of ErPCs with sirolimus. Only response-positive patients will be judged to be eligible to receive sirolimus treatment.
Inclusion criteria
Inclusion criteria were the following: (a) patients with confirmed genotypes of homozygous/compound heterozygous-thalassemia major (β+/β+, β+/β0, β0/β0 thalassemia genotype); (b) patients with documented diagnosis of transfusion dependence requiring more than 8 transfusion during the preceding 12 months; (c) patients on regular transfusion since at least 6 years; (d) patients with age over 18 years; (e) patients with splenectomy performed at least 60 days before selection or spleen dimensions < 20 cm in the longitudinal diameter as detected by abdominal echography; (f) a medically reliable method of contraception for the entire study duration must be counseled for female participants potential childbearing; (g) patients willing to follow all the study requirements and perform all the study visits and to cooperate with the investigator; (h) patient able to understand the informed consent and to sign it before any study procedure;
Exclusion criteria
Exclusion criteria were the following: (a) patients treated with hydroxyurea (HU) at selection visit or in the last 6 months; (b) ongoing treatment with drugs possibly affecting sirolimus actions, including treatment with macrolidic antibiotics (chlarithromycin); (c) cytotoxic agents, systemic corticosteroids, immunosuppressants or anticoagulant therapy such as warfarin or heparin within 28 days before inclusion (prophylactic aspirin up to 100 mg/day is allowed); (d) history of severe allergic or anaphylactic reactions or hypersensitivity to excipients in the experimental drug; (e) cardiovascular complications (heart failure) as classified by the New York Heart Association (NYHA) classification 3 or higher; uncontrolled hypertension defined as systolic blood pressure (BP) ≥ 140 mm Hg or diastolic BP ≥ 90 mm Hg; significant arrhythmia requiring treatment; QTc> 450 msec on selection ECG; ejection fraction <50% by echocardiogram, MUGA or cardiac magnetic resonance; myocardial infarction within 6 months prior of selection;); (f) coexisting viral infections (positivity for human immunodeficiency virus (HIV) antibody; active hepatitis B (HBV) or hepatitis C (HCV) as demonstrated by the presence of hepatitis B surface antigen (HBsAg) and a positive HCV-RNA test, HBcAb and HBV-DNA positivity); (g) white blood cell count <3000 cells/ uL and/or Granulocytes <1500/ uL; (h) platelet count <150.000/uL and >1 x106 /uL; (i) dyslipidemia (total cholesterol > 240 mg/dl; triglycerides > 200 mg/dl); (l) significant proteinuria (>1g/24 hrs); (m) increased levels of transaminases (more than 3 times the upper limit of normal); (n) major surgery (including splenectomy) within 60 days before selection (patients must have fully recovered from any previous surgery); (o) iron chelation therapy: Deferiprone is not accepted as a chelation therapy drug, while Desferioxamine and Deferasirox are allowed; (p) pregnant or lactating women; patients who are expecting to get pregnant during the next 12 months; (q) treatment with live vaccines within 90 days preceding the selection; (r) subject with history or current malignancies (solid tumors and hematological malignancies) or presence of masses/tumor detected by ultrasound at selection; (s) subject with any significant medical condition and/or laboratory abnormality considered by the investigator as not adequately controlled at the time of selection.
Subject Enrolment
All the patients who fulfill inclusion/exclusion selection criteria receive a patient information sheet to read and time to clarify doubts with investigators before consenting. Informed written consent from all participants is obtained before recruiting them into the study. At the time of enrolment, information on sociodemographic background, family history, medical history, present medical problems and concomitant medication are received and carefully considered. In the selection period of the study physical examination, cardiac evaluation, abdominal ultrasound and blood tests are performed. Summarizing, eligible for the study are patients who fulfill inclusion/exclusion criteria and who are responsive to sirolimus in vitro (ErPCs) according to laboratory specific definition (≥ 20% increase of HbF in comparison with samples not treated with sirolimus).
Intervention
The investigational drug in the form of coated tablets (0.5 mg sirolimus) is provided to the patients in blisters, adequately labelled with the information regarding the study. At visit 2 (V2, day 0), (Figure 2) patients will be instructed to take 2 tablets (0.5 mg each). The sirolimus blood level will be measured for the first time after 10 days of therapy and then on day 90 (visit 6, V6), on day 180 (visit 8, V8) and on day 360 (visit 11, V11). Treatment dose will be increased up to 2 mg/day or decreased to 0.5 mg/day in order to maintain sirolimus whole blood concentrations in a range between 5- 8 ng/mL. The dosage will be kept constant unless a reduction dictated by side effects. All other standard treatments, including blood transfusions and iron chelation will be continued. All patients will receive leuco-depleted packed red blood cell transfusions in order to maintain a pre-transfusion level of hemoglobin between 9-10.5 g/dL, strictly in agreement with the International Thalassaemia Federation Guidelines.
Study Procedure
Clinical evaluation
All patients will be reviewed regularly during the 360 days of the intervention period (visit 3-11) and followed-up for a further 3 months after discontinuation of treatment (visit 12, V12). During each review visit, a trained doctor will complete an interviewer to assess compliance with ongoing therapy, consumption of extra drugs, symptoms related to known and unknown adverse effects and tolerability of sirolimus. At each visit a complete physical examination will be done to assess weight, height, vital signs, adverse effect of sirolimus. The exact volume of transfused blood done during the treatment period and in the 180-360 days before the start of sirolimus will be recorded.
Compliance evaluation and quality of life assessment
Compliance on sirolimus intake will be assessed by interview and verified with returned drug accountability. At visits on day 0, 180 and 360, before proceeding with the clinical assessments, patients will be requested to complete TranQoL questionnaires.
Laboratory evaluation
The molecular evaluation of the α-globin and β-globin genotypes will be performed at enrolment to determine the molecular characteristics of the patients. For the assessment of efficacy and safety outcome the following laboratory tests will be performed during the study: (a) measurement of HbF levels in blood: at visits day 0, 10, 90, 180 and 360, through HPLC; (b) measurement of HbF and total hemoglobin production in ErPCs: at selection, day 0, day 90, day 180 and day 360 (c) measurement of γ-globin expression: globin gene expression will be analyzed by real time RT-qPCR at selection, day 0, day 90, day 180 and day 360; (d) analysis of biomarkers for erythropoiesis and hemolysis (reticulocytes count, nucleated red blood cells, serum lactate dehydrogenase, serum bilirubin level, erythropoietin level, serum transferrin receptor level): at visit day 0, 180, 360; (e) measurement of serum ferritin level: at day 0, 90, 180 and 360; (f) peripheral blood immunophenotype-Lymphocyte subsets measurement: Immunophenotype will be detected by FACS analysis, measuring CD3, CD4, CD8, Treg (CD4+CD25high), CD19 and CD14 at visit 0, 90 and 360 days; (g) immune functions analysis: Th1/Th2/Th17 cytokine detection will be used for the quantitative analysis of cytokines in the supernatants (IFNγ, TNF, IL-10, IL-5, IL-4 and IL-2, and IL-17) at visit 0, 90 and 360 days; (h) peripheral blood immunophenotype-Lymphocyte subsets measurement: evaluation of the expression of CD3, CD4, CD8, CD25, CD19 CD14 in PBMCs at day 0, 90, 360; (i) biochemical and hematological analysis (Full blood count, Reticulocytes count, Proteinuria, Creatinine, ɤ-GT, Alkaline phosphatase, AST, ALT, Total and indirect bilirubin, Glucose, Sodium, potassium, chloride, phosphorous, calcium , Albumin , Uric acid , Cholesterol, Triglycerides): at each study visit (except for day 10); (l) HBV-DNA test: only in patients HBcAb positive at selection, at visit 180 and 360 day.
Safety Evaluation
At follow-up visits during the intervention and 3-month post intervention periods, patients will be interviewed and examined by a trained doctor to monitor adverse effects of sirolimus treatment. Expected adverse events of sirolimus are reported in the "summary of product characteristics" of sirolimus. In UE, Rapamune is indicated for the prophylaxis of organ rejection in adult patients at low to moderate immunological risk receiving a renal transplant in combination with corticosteroids and/or ciclosporin, and for the treatment of patients with sporadic lymphangioleiomyomatosis with moderate lung disease or declining lung function.
In renal transplantation patients, blood levels of sirolimus should be maintained between 16 and 24 ng/mL, and the most common side effects (in more than 1 in 10 people) are thrombocytopenia, anemia, pyrexia, hypertension, hypokalemia, hypophosphatemia, urinary tract infection, hypercholesterolemia, hyperglycemia, hypertriglyceridemia, abdominal pain, lymphocele, peripheral oedema, arthralgia, acne, diarrhea, pain, constipation, nausea, headache, increased blood creatinine, and increased blood lactate dehydrogenase (LDH).
In patients with lymphangioleiomyomatosis, sirolimus therapy is suggested at lower dose (initial dose 2 mg/day, blood concentrations managed to be between 5–15 ng/mL) and the most common adverse reactions (included aphthous ulcers, diarrhea, and upper respiratory infections) are consistent with the safety profile of the product for the indication prophylaxis of organ rejection in renal transplantation.
During the clinical trial, adverse effects (AEs) resulting from any abnormal laboratory or instrumental finding, symptom or disease temporally associated with the use of the investigational product, will be reported; AEs will be classified as expected or un-expected event, on severity (serious, mild, moderate, severe according to the criteria of Common Terminology Criteria for Adverse Events Version 5.0) and whether or not related to the investigational product.
Transient suspension (in case of mild or moderate AE with possible/probable correlation with the drug) and definitive suspension (severe AE possibly related to sirolimus administration, or relapsing moderate AE) should be considered. Suspected adverse events will be reported according to the national guideline. Patients receive specific information to manage and prevent the adverse event more likely to be observed, in particular for the oral cavity toxicity.
Outcome Measures
Primary outcome
The primary outcome is the increase of HbF fraction in peripheral blood obtained at day 360 compared to day 0 (baseline).
Secondary outcome
The secondary outcomes are as follows. (a) The increase of HbF fraction in peripheral blood obtained at day 10, 90, 180 and 360 compared to day 0 (baseline); (b) the increase of HbF fraction and total hemoglobin production in ErPCs obtained at day 90, 180 and 360 compared to selection and day 0 (baseline); (c) the increase of globin gene expression obtained at day 90, 180 and 360 compared to selection and day 0 (baseline); (d) improvement of the biomarkers for erythropoiesis and hemolysis level obtained at day 180, 360 compared with day 0; (e) reduction in the number of red cell units and in the total blood quantity (in mL) transfused in semesters bfpre and under treatment (day -360 to -180, day -180 to 0, day 0 to 180, day 180 to 360); (f) change in the intake of iron chelators at days 180 and 360 compared to baseline; (g) change in serum ferritin level at day 90, 180 and 360 in comparison with day 0; (h) change in the peripheral blood immunophenotype-Lymphocyte subsets and quantitative analysis of IgG/IgA/IgM at day 90 and 360 compared to day 0; (i) change in the quality of life at 6 and 12 months compared to baseline through TranQoL questionnaire; (l) quantitative analysis of cytokines (IFNγ, TNF-alpha, IL-10, IL-5, IL-4 and IL-2, and IL-17) released by supernatants of peripheral blood mononuclear cells (PBMC) isolated from treated patients at day 90 and 360 compared to day 0; (m) change in the expression of CD3, CD4, CD8, CD25, CD19 CD14 in PBMCs at day 90 and day 360 compared to day 0; (n) occurrence of abnormal biochemical and hematological parameters during the study visits compared to day -40 or day 0; (o) incidence of the side effects of sirolimus at each study visit starting from day 10 visit; (p) change in the cardiovascular function (ECG, compared at day 180 and final visit vs selection visit; echocardiography, compared at final visit vs selection visit); (q) change in spleen dimensions though abdominal ultrasound examination compared at 180 days and at final visit vs selection visit; (r) occurrence of HBV-DNA positivity in patients with HBcAb positive at selection visit at day 180 and day 360 visits.
Data management and monitoring
Electronic CRF (e-CRF) system will be used for the collection of source data. All data entered in the system will be anonymized and patients will be identified only through the study number. Database is protected by a daily backup and is not accessible from external remote systems but only from local application. Only database administrator (DBA) can access to data using a crypted connection to the server. Data will be directly exported by DBA, in the format needed by the Statistician. After statistical analysis, an offline copy of whole database will be saved in a crypted file, which will be stored according to law and guidelines. The study will be monitored by means of on-site visits and regular inspections of the CRF. The biological material will be used for protocol related analysis and will be destroyed after the publication of the study data. The composition of the Data Safety Monitoring and of the Scientific Committee comprised independent member such as hematologist, clinical pharmacologist, nephrologist and patient’s association expert in order to make appropriate recommendations on participant safety and trial integrity.
Ethical considerations
Participants will have the right to withdraw from the trail at any point without providing explanations.
Approval of the study indicated as Sirthalaclin has been obtained from the Ethic Committee Area Vasta Emilia Romagna Centro (Ferrara) (January 23, 2019). Approval for the study indicated as Thala-Rap has been obtained from: (a) the Ethic Committee Area Vasta Emilia Romagna Centro (Ferrara) (April 17, 2019); the Ethic Committee Regione Toscana Area Vasta Nord (Pisa) (February 5, 2020); the Ethic Committee for Clinical Experimentation of Regione Toscana (Firenze) (February 25, 2020).
Termination of the trial
The Sponsor, the investigator and any competent authority may terminate the study prematurely at any time when any of the below circumstances is detected: (a) incidence or severity of adverse events which indicates a potential health hazard to the patients; (b) new scientific knowledge or other information available which indicates that continuation of the study would place the patients at undue risk; (c) any other safety concern. The trials will be concluded unless > 25% of participants will be permanently discontinued due to safety concern. In addition, the DSMC could recommend an early termination of the trial to the Executive Committee who will take the final decision in collaboration with the Sponsor. In case of early study termination, a written confirmation will be prepared by the Sponsor and will be dated and signed by the investigators. The Ethics Committees and Competent Authorities will be informed according to local regulations
Conclusions
After completion of the two trials previously described the perspective for clinical development of sirolimus in β-thalassemia will be clearer. First of all, tolerability of the drug in these patients will be evaluated. Even if the small number of patients may not be adequate for the evaluation of a change in transfusion requirement in transfusion dependent patients, the changes in biomarkers related to erythropoiesis and hemoglobin status may be of relevance for the design of future trials in various patients with hemoglobin disorders.
Acknowledgments
The sponsors of the trials are Rare Partners srl Impresa Sociale (Sirthalaclin) and the Department of Life Sciences and Biotechnology, Ferrara University (Thala-Rap). Pfizer Italy is supplying free of charge Rapamune tablets for the Thala-Rap trial. Supported by Wellcome Trust (United Kingdom, Innovator Award 208872/Z/17/Z); by the UE FP7 THALAMOSS Project (THALAssaemia MOdular Stratification System for personalized therapy of β-thalassemia; grant n.306201-FP7-Health-2012-INNOVATION-1) and by AIFA (Agenzia Italiana del Farmaco, Italy, AIFA-2016-02364887). The scientific activity of M.R.G and R.G. is supported by Associazione per la Lotta alla Talassemia di Ferrara (A.L.T.).
Disclaimer
The funding bodies had no role in the design or conduct of the study, collection, management, analysis and interpretation of the data, or preparation, review and approval of the manuscript.
Competing Interests
The authors declare that they have no competing interests. M.P. is the administrator of “Rare Partners srl Impresa Sociale”, who licensed the rights for the patent WO 2004/004697 on the use of sirolimus in -thalassemia.
Abbreviations
Hb: hemoglobin; HbA: adult hemoglobin; HbF: fetal hemoglobin; HPFH: hereditary persistence of fetal hemoglobin; TDT: transfusion-dependent thalassemia; SCD, sickle-cell disease; HU: hydroxyurea; mTOR: mammalian target of rapamycin; ROS: reactive oxygen species; hHSPCs: Human Hematopoietic Stem and Progenitor Cells; ErPCs: erythroid precursor cells; SLE: systemic lupus erythematosus; LAM: lymphangioleiomyomatosis; HIV: human immunodeficiency virus; HBV: hepatitis B virus; HCV: hepatitis C virus; FACS: fluorescence-activated cell sorter; NGS: next-generation sequencing; AEs: adverse effects; EU: European Union; ODD: orphan drug designation, ODD; EMA: European Medicinal Agency; FDA: Food and Drug Administration.
References
1. Weatherall DJ. Phenotype-genotype relationships in monogenic disease: lessons from the thalassaemias. Nat Rev Genet. 2001; 2(4):245-55.
2. Origa R. β-Thalassemia. Genet Med. 2017; 19: 609-619.
3. Fucharoen S, Weatherall DJ. Progress Toward the Control and Management of the Thalassemias. Hematol Oncol Clin North Am. 2016; 30: 359-71.
4. Modell B, Darlison M, Birgens H, et al. Epidemiology of haemoglobin disorders in Europe: an overview. Scand J Clin Lab Invest. 2007; 67:39-69.
5. Galanello R, Origa R. Β-thalassemia. Orphanet J Rare Dis. 2010; 5:11.
6. Colah R, Gorakshakar A, Nadkarni A. Global burden, distribution and prevention of β-thalassemias and hemoglobin E disorders. Expert Rev Hematol. 2010; 3:103-17.
7. Modell B, Darlison M. Global epidemiology of haemoglobin disorders and derived service indicators. Bull World Health Organ. 2008; 86:480-7.
8. Thein SL. The molecular basis of β-thalassemia. Cold Spring Harb Perspect Med. 2013; 3: a011700
9. Orkin SH, Kazazian HH Jr, Antonarakis SE, et al. Abnormal RNA processing due to the exon mutation of β E-globin gene. Nature. 1982; 300:768-769
10. Olivieri NF, Pakbaz Z, et al. Hb E/β-thalassaemia: a common & clinically diverse disorder. Indian J Med Res. 2011; 134:522-531
11. Pavani G, Fabiano A, Laurent M, et al. Correction of β-thalassemia by CRISPR/Cas9 editing of the α-globin locus in human hematopoietic stem cells.
Blood Adv. 2021; 5:1137-1153.12. Mettananda S, Fisher CA, Hay D, et al. Editing an α-globin enhancer in primary human hematopoietic stem cells as a treatment for β-thalassemia. Nat Commun. 2017; 8:424.
13. Sripichai O, Fucharoen S. Fetal hemoglobin regulation in β-thalassemia: heterogeneity, modifiers and therapeutic approaches. Expert Rev Hematol. 2016; 9:1129-1137.
14. Liu D, Zhang X, Yu L, et al. KLF1 mutations are relatively more common in a thalassemia endemic region and ameliorate the severity of β-thalassemia. Blood. 2014; 124:803–811
15. Forget BG. Molecular basis of hereditary persistence of fetal hemoglobin. Ann N Y Acad Sci. 1998; 850:38-44.
16. Musallam KM, Sankaran VG, Cappellini MD, et al. Fetal hemoglobin levels and morbidity in untransfused patients with β-thalassemia intermedia. Blood. 2012; 119:364-367.
17. Nuinoon M, Makarasara W, Mushiroda T, et al. A genome-wide association identified the common genetic variants influence disease severity in β0-thalassemia/hemoglobin E. Hum Genet. 2010; 127:303-314.
18. Galanello R, Sanna S, Perseu L, et al. Amelioration of Sardinian β0 thalassemia by genetic modifiers. Blood. 2009; 114:3935-3937.
19. Danjou F, Anni F, Perseu L, Satta S, Dessì C, Lai ME, Fortina P, Devoto M, Galanello R. Genetic modifiers of β-thalassemia and clinical severity as assessed by age at first transfusion. Haematologica. 2012; 97:989-993.
20. Badens C, Joly P, Agouti I, et al. Variants in genetic modifiers of β-thalassemia can help to predict the major or intermedia type of the disease. Haematologica. 2011; 96: 1712-1714.
21. Uda M, Galanello R, Sanna S, et al. Genome wide association study shows BCL11A associated with persistent fetal hemoglobin and amelioration of the phenotype of β-thalassemia. Proc Natl Acad Sci USA. 2008; 105:1620-1625.
22. Gambari R, Fibach E. Medicinal chemistry of fetal hemoglobin inducers for treatment of β-thalassemia. Curr Med Chem. 2007; 14:199-212.
23. Lavelle D, Engel JD, Saunthararajah Y. Fetal Hemoglobin Induction by Epigenetic Drugs. Semin Hematol. 2018; 55:60-67.
24. Finotti A, Gambari R. Recent trends for novel options in experimental biological therapy of β-thalassemia. Expert Opin Biol Ther. 2014; 14:1443-54.
25. Antoniani C, Meneghini V, Lattanzi A, et al. Induction of fetal hemoglobin synthesis by CRISPR/Cas9-mediated editing of the human β-globin locus. Blood. 2018; 131:1960-1973.
26. Wu Y, Zeng J, Roscoe BP, et al. Highly efficient therapeutic gene editing of human hematopoietic stem cells. Nat Med. 2019; 25:776-783.
27. Métais JY, Doerfler PA, Mayuranathan T, et al. Genome editing of HBG1 and HBG2 to induce fetal hemoglobin. Blood Adv. 2019; 3:3379-3392.
28. Mingoia M, Caria CA, Ye L, et al. Induction of therapeutic levels of HbF in genome-edited primary β(0) 39-thalassaemia haematopoietic stem and progenitor cells. Br J Haematol. 2021; 192:395-404.
29. Frangoul H, Altshuler D, Cappellini MD, et al. CRISPR-Cas9 Gene Editing for Sickle Cell Disease and β-Thalassemia. N Engl J Med. 2021; 384:252-260.
30. NCT03655678 (A Safety and Efficacy Study Evaluating CTX001 in Subjects With Transfusion-Dependent β-Thalassemia)
31. Sehgal SN. Sirolimus: its discovery, biological properties, and mechanism of action. Transplant Proc. 2003; 35(3 Suppl):7S-14S.
32. Kahan BD. Sirolimus: a new agent for clinical renal transplantation. Transplant Proc. 1997; 29: 48-50.
33. Vasquez EM. Sirolimus: a new agent for prevention of renal allograft rejection. Am J Health Syst Pharm. 2000; 57:437-48.
34. Hernández D, Martínez D, Gutiérrez E, et al. Clinical evidence on the use of anti-mTOR drugs in renal transplantation. Nefrologia. 2011; 31:27-34.
35. Schaffer SA, Ross HJ. Everolimus: efficacy and safety in cardiac transplantation. Expert Opin Drug Saf. 2010; 9:843-54.
36. Tang CY, Shen A, Wei XF, et al. Everolimus in de novo liver transplant recipients: a systematic review. Hepatobiliary Pancreat Dis Int. 2015; 14:461-9.
37. Ji L, Xie W, Zhang Z. Efficacy and safety of sirolimus in patients with systemic lupus erythematosus: A systematic review and meta-analysis. Semin Arthritis Rheum. 2020; 50:1073-1080.
38. Wang Q, Luo M, Xiang B, et al. The efficacy and safety of pharmacological treatments for lymphangioleiomyomatosis. Respir Res. 2020; 21:55.
39. Sasongko TH, Ismail NF, Zabidi-Hussin Z. Rapamycin and rapalogs for tuberous sclerosis complex. Cochrane Database Syst Rev. 2016; 7:CD011272.
40. Graillon T, Sanson M, Campello C, et al. Everolimus and Octreotide for Patients with Recurrent Meningioma: Results from the Phase II CEVOREM Trial. Clin Cancer Res. 2020; 26:552-557.
41. Gallo M, Malandrino P, Fanciulli G, et al. Everolimus as first line therapy for pancreatic neuroendocrine tumours: current knowledge and future perspectives. J Cancer Res Clin Oncol. 2017; 143:1209-1224.
42. Manohar PM, Beesley LJ, Taylor JM, et al. Retrospective Study of Sirolimus and Cyclophosphamide in Patients with Advanced Differentiated Thyroid Cancers. J Thyroid Disord Ther. 2015; 4:188.
43. Hortobagyi GN. Everolimus plus exemestane for the treatment of advanced breast cancer: a review of subanalyses from BOLERO-2. Neoplasia. 2015; 17:279-88.
44. Merli M, Ferrario A, Maffioli M, et al. Everolimus in diffuse large B-cell lymphomas. Future Oncol. 2015; 11:373-83.
45. Motzer RJ, Escudier B, Oudard S, et al. Phase 3 trial of everolimus for metastatic renal cell carcinoma: final results and analysis of prognostic factors. Cancer. 2010; 116:4256-65.
46. Mischiati C, Sereni A, Lampronti I, et al. Rapamycin-mediated induction of gamma-globin mRNA accumulation in human erythroid cells. Br J Haematol. 2004; 126:612-21.
47. Fibach E, Bianchi N, Borgatti M, et al. Effects of rapamycin on accumulation of alpha-, β- and gamma-globin mRNAs in erythroid precursor cells from β-thalassaemia patients. Eur J Haematol. 2006; 77:437-41.
48. Zuccato C, Bianchi N, Borgatti M, Lampronti I, Massei F, Favre C, Gambari R. Everolimus is a potent inducer of erythroid differentiation and gamma-globin gene expression in human erythroid cells. Acta Haematol. 2007; 117:168-76.
49. Pecoraro A, Troia A, Calzolari R, et al. Efficacy of Rapamycin as Inducer of Hb F in Primary Erythroid Cultures from Sickle Cell Disease and β-Thalassemia Patients. Hemoglobin. 2015; 39:225-9.
50. Ansari SH, Lassi ZS, Khowaja SM, et al. Hydroxyurea (hydroxycarbamide) for transfusion-dependent β-thalassaemia. Cochrane Database Syst Rev. 2019; 3:CD012064.
51. Algiraigri AH, Wright NAM, Paolucci EO, et al. Hydroxyurea for lifelong transfusion-dependent β-thalassemia: A meta-analysis. Pediatr Hematol Oncol. 2017; 34:435-448.
52. Algiraigri AH, Kassam A. Hydroxyurea for hemoglobin E/-thalassemia: a systematic review and meta-analysis. Int J Hematol 2017; 106:748–56.
53. Foong WC, Ho JJ, Loh CK, Viprakasit V. Hydroxyurea for reducing blood transfusion in non-transfusion dependent β thalassaemias. Cochrane Database Syst Rev. 2016; 10: CD011579.
54. Bianchi N, Finotti A, Ferracin M, et al. Increase of microRNA-210, decrease of raptor gene expression and alteration of mammalian target of rapamycin regulated proteins following mithramycin treatment of human erythroid cells. PLoS One. 2015; 10(4):e0121567.
55. Finotti A, Bianchi N, Fabbri E, et al. Erythroid induction of K562 cells treated with mithramycin is associated with inhibition of raptor gene transcription and mammalian target of rapamycin complex 1 (mTORC1) functions. Pharmacol Res. 2015; 91:57-68.
56. Zhang X, Campreciós G, Rimmelé P, et al. FOXO3-mTOR metabolic cooperation in the regulation of erythroid cell maturation and homeostasis. Am J Hematol. 2014; 89:954-63.
57. Khaibullina A, Almeida LE, Wang L, et al. Rapamycin increases fetal hemoglobin and ameliorates the nociception phenotype in sickle cell mice. Blood Cells Mol Dis. 2015; 55:363-72.
58. Wang J, Tran J, Wang H, et al. mTOR Inhibition improves anaemia and reduces organ damage in a murine model of sickle cell disease. Br J Haematol. 2016; 174:461-9.
59. Lechauve C, Keith J, Khandros E, et al. The autophagy-activating kinase ULK1 mediates clearance of free α-globin in β-thalassemia. Sci Transl Med. 2019; 11(506):eaav4881.
60. Gaudre N, Cougoul P, Bartolucci P, et al. Improved Fetal Hemoglobin With mTOR Inhibitor-Based Immunosuppression in a Kidney Transplant Recipient With Sickle Cell Disease. Am J Transplant. 2017; 17:2212-2214.
61. Al-Khatti AA, Alkhunaizi AM. Additive effect of sirolimus and hydroxycarbamide on fetal haemoglobin level in kidney transplant patients with sickle cell disease. Br J Haematol. 2019; 185: 959-961.
Received: May 08, 2021;
Accepted: June 09, 2021;
Published: June 13, 2021.
To cite this article : Gamberini MR, Prosdocimi M,Roberto Gambari R. Sirolimus For Treatment of β-Thalassemia: From Pre-Clinical Studies To The Design Of Clinical Trials. Health Education and Public Health. 2021; 4:3.
© 2021 Gamberini MR, et al.